Scientific Basis of Active Isolated Stretching: A Review Pertti T. Kukkonen
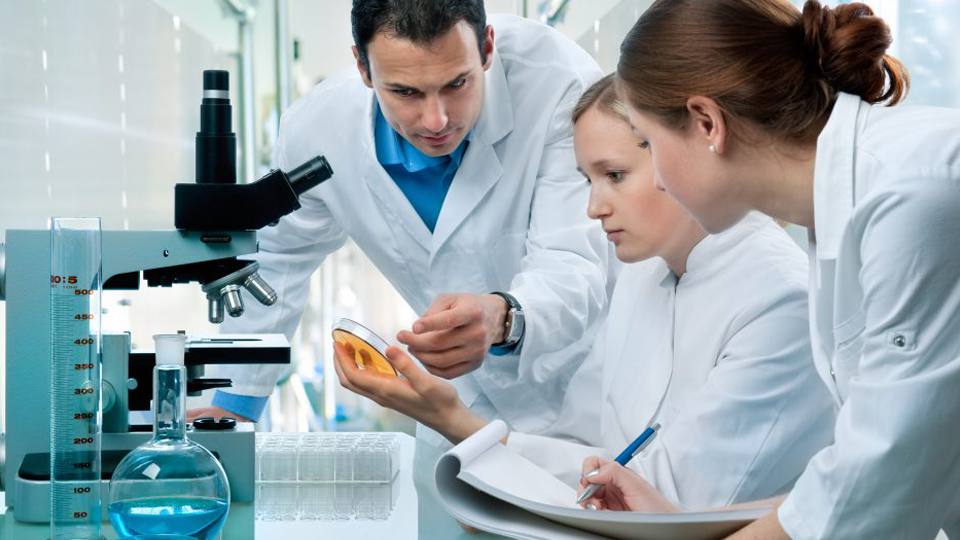
Pertti T. Kukkonen
Research Director, Espoo, Finland
ABSTRACT
Kukkonen PT. Scientific Basis of Active Isolated Stretching: A
Review. JEPonline 2019(22)2:58-70. Aaron L. Mattes made the
observation that stretching along the line of stress of the muscle
and relaxation of the muscle in each repetition of stretch would
diminish the resistance to stretching. Based on this observation,
the purpose of this review will be to demonstrate that Active
Isolated Stretching (AIS) is performed with less torque than static
stretches, but nonetheless increases joint range of movement
(ROM) of the hamstring muscles more so than static stretches.
By searching the studies on static stretching, it was possible to
find 14 articles that reported mean maximal torques of hamstring
and calf muscles. These measurements were compared to
optimal – maximal torque of AIS. The result is that the AIS
stretches are performed with less torque than static stretches.
This inference should be confirmed by experimental studies. It
was also possible to find 4 articles that reported AIS increasing
ROM of the hamstring muscles more or at least the same
amount as static stretching. Mattes finding, that stretching along
the line of stress of the muscle and relaxation of muscle in each
repetition is the main mechanism of action of AIS. The findings of
this review would be the basic theory of AIS. The main
mechanism of AIS could renew the stretching techniques.
Hence, this review should have very positive effect on exercise
physiology.
Key Words: Flexibility, Musculoskeletal, Relaxation
59
INTRODUCTION
Few exercise physiology research studies have been published on Active Isolated
Stretching (AIS). The mechanism of action of AIS has not been thoroughly investigated.
On some other stretching techniques, Weppler and Magnusson (31) published in 2010
a review of the mechanisms of action of the techniques. This development was made
possible by evaluating the biomechanical properties of stretching. When including the
use of tension in muscle length evaluation, studies on stretching were able to construct
torque-angle curves before and after stretching. Measuring maximal torques used in
most stretching techniques became possible.
Mattes (20-23) was developing AIS when he was directing Kinesiotherapy Clinics at the
University of Illinois in 1972-1976 and at the University of Toledo in 1976-1979. He is an
expert in massage therapy and kinesiotherapy. As an athlete himself, he was frustrated
when he suffered an injury and there were only ineffective rehabilitation methods
available. By trying a great number of positions and stretches, he finally found a
stretching technique that seemed to function properly. Mattes found in his many trials
the combination of stretching along the stress line of the muscle and relaxation of the
muscle to be stretched in each of the repetitions. Both were needed in order to diminish
the resistance to stretching of a muscle.
If the resistance to stretching is smaller along stress lines, why have the numerous
researchers not discovered, even by accident, to use stress line of muscle when
investigating static stretching? If the muscle is not relaxed, stretching along the stress
line does not diminish the resistance. Relaxation could be a precondition for smaller
resistance.
According to Mattes (21), AIS is performed very lightly, with less than 1 lb (4.5 Nm)
pressure. The optimum pressure is only 6 to 8 ounces or 1.7 to 2.3 Nm (30). Stretch
lasts 1 to 2 sec and there are 8 to 10 reps of stretch. The muscle to be stretched is
relaxed by contracting the antagonist muscle when moving body part actively from the
starting position to the stretching position.
Mattes would have been developing AIS gradually and finally in books of 2000 (20,23)
and 2012 (21) he concluded that the laws of Sherrington and Wolff formed the scientific
basis of AIS (20,21). But, since this was not convincing evidence of the scientific value
of AIS, he tried to get medical researchers involved in developing AIS. According to
“Massage Magazine” (internet), Mattes and his associate, Jeffrey Haggquist DO,
arranged in Washington D.C. in June of 2008 from the 10th to the 14th a seminar,
where AIS and medical experts together developed AIS applications. The aim was also
to encourage researchers to start researching AIS. In 2009, Mattes was working with
NIH (National Institutes of Health) to design pilot studies to demonstrate the value of
AIS to the scientific community (30).
These attempts did not succeed because the researchers at that time did not have the
tools to show the value of AIS. Before the year 2010 only a few research teams were
60
able to use torque-angle curves and maximal torques in stretching and had devices to
measure them. The main interest of these research teams was in sensory theory of
static stretch and not in AIS. Since Weppler and Magnusson`s review article (31) in
2010, torque-angle curves and maximal torques in stretching have been better known.
SOME IDEAS OF AARON L. MATTES
Relaxation
Mattes (21) states that only relaxed myofascial structures can be optimally stretched.
Relaxation is based on Sherrington`s law of reciprocal inhibition. Contraction of an
agonist muscle on one side of a joint sends a signal to the opposite side muscle to
relax. The positions and movements of AIS are designed so that the movement of
stretching a person from a starting position to a stretching position relaxes the muscle or
muscles automatically. Relaxation is augmented by exhaling of the person when moving
through the stretching position. After each stretch, the area being stretched should
always be returned to the starting position before the next repetition. There should be 8
to 10 reps for each stretch.
Stretching Along the Lines of Stress in the Body and Muscle
Stretching should be directed along the stress line for each muscle. Mattes derived this
principle from Wolff`s law that originally concerns bones. Also, the sheets of fascia are
laid down along the lines of stress within the body and they adhere to proper anatomical
positioning. The conclusion by Mattes (21) is supported by Myers (26) who
demonstrated how long collagen molecules of muscle orient themselves along the lines
of tension of the muscle. Mattes (21) expressed the same anatomical feature on macro
level with the collagen fibres laid down along the lines of stress within the body.
Mattes studied origin, insertion, and action of each muscle to decide the optimal
direction it should be stretched. Parts of some muscles have their own stress lines and
optimal stretches. Stretching along the line of stress of the muscle and relaxation of
muscle minimizes tension and friction among fascial sheets so that they start to move,
although stretch is done with minimal pressure or torque. In repetitions of the stretch,
this works to break down adhesions and scar tissue formations that have been caused
by inflammation resulting from soft tissue traumas. Breaking down adhesions mitigates
or removes muscular pain. Stretches also realign collagen fibres and reduce muscle
spasms.
Repetitive Muscle Contractions and Breathing in Their Rhythm
In order to facilitate relaxation, one should exhale when moving from starting position to
stretching position and inhale when returning back to starting position. Breathing and
repetitive muscle contractions deliver greater amounts of circulating blood, oxygen, and
nutrition to the muscles. They also stimulate circulation and drainage of lymph, which
helps to eliminate metabolic wastes, such as lactic acid. Joint angle expands in each
repetition. Combining flexibility and strength training systems is one feature of AIS.
Muscle opposite to the one being stretched is being strengthened at the same time.
This takes place in each repetition. Movement increases strength of muscles in good
61
balance on both sides of the body, as well on both sides of the lower and upper
extremities when all major muscles are stretched systematically.
COMPARISON OF THE MAXIMAL TORQUES IN AIS AND STATIC STRETCHING
Because no studies have been published on the mechanisms of action of AIS in peerreviewed
journals, a Master of Science thesis in kinesiology at the Brock University in
Ontario, Canada, has been used (for one part) in the comparison. The title of the study
is “Active Isolated Stretching: An Investigation of the Mechanical Mechanisms” by
Longo (14). The experimental part of the study provides some valuable hints on the
mechanisms of action of AIS. The subjects in the study were university students (8
females and 2 males) with tight hamstrings. During 6 wks they made daily 2 series of
knee (right side) extension AIS stretches with 10 reps with a 30-sec interval between
series. The stretches were done with less than 1 lb force and the last (10th) repetition
was taken so far that the subject felt a light irritation. The left leg was a control. Before
and after the 6 wks of stretching, knee extension ROM and resistance to stretch were
measured with a dynamometer (Biodex System 3) for both legs (with gravity correction).
The Delsys Bagnoli-4EMG-system was used to monitor electric activity of the vastus
lateralis and hamstring muscle group with electrodes placed on the muscles.
Main result of Longo`s experiment was that the mechanism of action of AIS is not
mechanical. Values measured before and after stretching are approximately on the
same line (Figure 1). Knee extension ROM increased significantly by 15° and there was
an indication that long-term AIS (6 wks in the experiment) would be efficient in
increasing ROM. Longo also made the conclusion that EMG measurements show that
the hamstring muscles were significantly less active than the vastus lateralis muscle
and that reciprocal inhibition was occurring and hamstrings were relaxed (14 p. 67).
Figure 1 is a modified figure from Longo`s study on knee extension AIS-stretching (14).
The person was applying only 30 Nm torque to do the final repetition of knee extension
AIS stretch. Unfortunately, mean maximal torque after stretching for all the 10 subjects
is not available. According to Mattes (30), the optimal AIS stretch is very light, about 2
Nm and with 10 reps at a torque of 20 Nm. The upper limit for the use of force is less
than 1 lb (or 4.5 Nm) torque. Therefore, for AIS optimal – maximal torque range is 20 to
45 Nm for comparison with static stretching.
62
Last column of Table 1 gives a list of mean maximal torques used in static stretches of
hamstring and calf muscles. The first 5 studies are found as references in Weppler and
Magnusson`s review article (30). The rest of the 9 studies are found from a review by
Freitas et al. (6) published in 2018. The review had the restriction in searching of
articles that only stretching interventions of more than 2 wks duration were accepted.
Table 1. A List of Mean Maximal Torques used in Static Stretches.
Studies of Static Stretching Increase Mean Maximal
Stretching Exercise in ROM Torque
weeks degrees Nm
Hamstring Muscles
Magnusson et al. (16) — — 75
Reid and McNair (28) 6 10 114
Ylinen et al. (32) 4 17 105
Ben and Harvey (2) 4 10 65
Folpp et al. (5) 4 9 67
Magnusson et al. (17) 3 10 52
Gajdosik (7) 3 13 50
Law et al. (12) 3 10 44
Chan et al. (4) 4 11 28
LaRoche & Connolly (11) 4 9 149
Calf Muscles
Gajdosik et al. (8) 8 5 122
Guissard & Duchateau (10) 6 8 29
Gajdosik et al. (9) 6 7 26
Blazevich et al. (3) 3 8 110
63
According to the studies in Table 1, mean maximal torques of static stretching range
between 26 and 149 Nm. For AIS the optimal-maximal range of torque would be 20 to
45 Nm. Although there is slight overlap in the ranges in Figure 2, one can infer that with
a quite high probability Figure 2 demonstrates that AIS is performed with less torque
than static stretch. The inference is reasonable, and it can be confirmed by studies
whereby the subjects are performing AIS and static stretches and torque-angle
relationships are measured as well as mean maximal torques after stretching. The
studies should be made both for hamstring muscles and calf muscles using available
measurement devices.
Table 2. Gender of Subjects has an Influence on Mean Maximal Torque.
Studies of Static Mean Maximal Subjects in
Stretching Torque Stretch Group
Nm Males Females
Hamstring Muscles
Magnusson et al. (16) 75 10 0
Reid and McNair (28) 114 23 0
Ylinen et al. (32) 105 12 0
Ben and Harvey (2) 65 9 21
Folpp et al. (5) 67 8 12
Magnusson et al. (17) 52 0 7
Gajdosik (7) 50 12 0
Law et al. (12) 44 15 15
Chan et al. (4) 28 6 4
LaRoche and Connolly (11) 149 9 0
Calf Muscles
Gajdosik et al. (8) 122 0 10
Guissard and Duchateau (10) 29 8 4
Gajdosik et al. (9) 26 0 6
Blazevich et al. (3) 110 12 0
Gender and age seem to influence the results of stretching, mainly because females are
more flexible than males and because flexibility is decreased after a certain age. In the
studies, males and aging subjects seem to use greater maximum torque than females
and younger persons. In the LaRoche and Connolly (11) study, the average age of the 9
participating men was 31 yrs of age, and some of them may have been over 60 yrs of
age. Therefore, they are less flexible and the mean maximal torque is high, 149 Nm.
The other high mean maximal torque of 122 Nm is in the Gajdosik et al. (8) study on calf
muscles of older women with an average age of 73 yrs. A very different story is reported
64
in Chan et al. (4) where the subjects are young women and men with an age range of
18 to 25 yrs and mean maximal torque is only 28 Nm.
THE MECHANISM OF ACTION OF AIS
The main mechanism of action of AIS would be stretching along the lines of stress in
the muscle and relaxation of the muscle to be stretched in each of the 8 to 10 reps.
Mattes and several AIS experts have a 40-yr experience in applying AIS in sport, sport
injuries, and various musculoskeletal problems. This means that there is a large
experience-based knowledge behind the other suggested mechanisms of action of AIS.
As a consequence of the main mechanism, friction among fascial sheets is reduced and
they start to move. This movement among fascial sheets during repetitions breaks down
adhesions and scar tissue formations of muscles. The breaking down of adhesions
mitigates or removes muscular pain. This is the first suggested mechanism, which
should be confirmed by more research in this area.
Breathing and repetitive muscle contractions accelerate circulation of blood and lymph.
This delivers oxygen and nutrition to muscles and reduces metabolic wastes like lactic
acid. The repetitive muscle contractions also work as strength training. These would be
the second suggested mechanism. Again, more research studies is essential to confirm
the suggested mechanisms. There might be other effects of AIS, such as stretching
reducing muscle spasms and realign collagen fibers as Mattes has suggested.
Therefore, the two suggested mechanisms may be just the beginning of the list of
possible mechanisms of AIS action.
ONE ASSUMPTION OF AIS SHOULD BE REVISED
Magnusson (18) review published in 1998 concluded that contractile reflex activity does
not contribute to the response in a slow static stretch. Magnusson`s conclusion is valid
also in regards to AIS. Alter (1) in his “Science of Flexibility” published in 2004 was
strongly critical of the assumption of the stretch reflex in AIS (1 p.164). Taking into
account these critics, the assumption of AIS, that the stretch reflex is activated if the
stretch continues more than 2 sec, should be revised. It could be revised without any
change in the way stretches are made. The change would only affect the way AIS is
justified. It has been demonstrated above that the best justification for AIS is stretching
along the stress lines of body and relaxation of the muscle to be stretched. Even in the
future, AIS-stretches would be performed in 2 sec with low force and by the rhythm of
breathing.
After the revision suggested above there would be no changes in the way AIS stretches
are done. Therefore, experimental studies on the effect of AIS on increasing joint ROM
would need no changes. Nor would there be any changes in the many ways AIS is used
in sports. The warning that one should not stretch more than 2 sec is in fact useless and
incorrect theoretical justification for AIS. To be better approved and appreciated, the
revision is inevitable.
65
WOULD AIS INCREASE JOINT ROM MORE THAN STATIC STRETCHING?
Four studies on this subject were published in peer-reviewed journals. They fulfil the
criteria of a scientific study. The studies have a randomized control group in addition to
the two groups doing the AIS and static stretches. Randomized assignment of the
subjects to the groups was secured. The studies were published as one page abstracts,
which may reflect the somewhat poor status of AIS in sports medicine and exercise
physiology.
1. McMahon et al. (24) evaluated 53 subjects who performed knee extension
stretches 4 times∙wk-1 for 4 wks. The increase in ROM was statistically significant
after the 1st wk of AIS and in static stretches after 2 to 4 wks. The AIS increase
in ROM was significantly greater than for the static stretch exercise.
2. Liemohn et al. (13) studied 30 subjects who performed 9 exercises of straight leg
raise in 3 wks. Both modes of stretching significantly increased ROM, but AIS
increased ROM significantly more than the static stretch exercise.
3. Marino et al. (19) evaluated 30 subjects who were doing the straight leg raise
stretch exercise 3 times∙wk-1 for as long as 13 wks. They reported that only the
AIS significantly increased ROM. As to the static stretch increase in ROM, it was
not significantly different from the control group.
4. Middag and Harmer (25) reported on 30 subjects who performed 5 times∙wk-1 for
3 wks knee extension stretches. AIS increased ROM more than 11% versus the
8% by static stretch, but the difference was not statistically significant.
In 2 of these 4 studies, AIS increased joint ROM significantly more than static stretch. In
the Middag and Harmer`s (25) study, AIS increased more but the difference was not
significant, and in Marino et al. (19) study, only AIS significantly increased ROM. Hence,
the studies point out that AIS attains greater or at least the same increase in joint angles
of the hamstring muscles than static stretch.
In the Lopez-Bedoya et al. (15) study, the findings indicated that static stretch increased
the hamstring ROM more than AIS and more than hold-relax stretching. However, AIS
was not correctly defined in the study, and the study procedures did not follow the ideas
of Mattes book published in 2000, although the book was in the reference list of the
study. Relaxation of the hamstring muscles in AIS exercises is specified incorrectly. The
authors are suggesting that assisted AIS is the same as assisted active static stretches
(AASS) with a 2-sec rest between repetitions and that during this 2-sec rest the muscle
is relaxing. For example, “Training session was thus: 4 x 12 x (AASS 2 sec) with a 2-sec
rest between repetitions and a 50-sec rest between series.” Relaxation of hamstrings is
not taking place in this procedure. Hamstrings are truly relaxed in AAIS (assisted AIS),
66
where the stretching person is actively raising the leg as far as possible, and the
assisting therapist is only finishing the stretch lightly, with less than 4.5 Nm torque.
Since relaxation of the muscle does not happen in AIS exercises in the study, one
essential part of the effect of AIS on ROM is missing and, therefore, the conclusion of
the study is not valid. AIS might as well be increasing ROM more than static stretch, but
the study is not able to give the correct estimate. Same authors, this time in a different
order, Vernetta-Santana et al. (29) published in 2015 another study on AIS. The study
sets out to confirm that AIS will significantly increase joint ROM, but will not weaken
significantly peak isometric force of the hamstring muscles. Definition of AIS is the same
as in the previous Lopez-Bedoyan et al. (15) study with the same incorrect specification
of relaxation of muscles. Therefore, the conclusions of the study are not likely to be
reliable.
DISCUSSION
Active Isolated Stretching has been developed for more than 40 yrs, but its scientific
basis has probably not been demonstrated until the earlier attempts by Mattes to get the
research community to study AIS. But that there are still concerns as explained earlier.
The suggested mechanisms of action of AIS should undergo further studies. After
possible confirmation by research, these mechanisms would be a valuable tool of AIS in
rehabilitation after sport injuries.
In fact, in this regards, Mattes made a 40-yr effort in developing and studying ~120
stretches for his 2012 book on AIS. The number of stretches is large and can serve the
needs of rehabilitation in physiotherapy. The book has instructions both for stretches
made by the client or patient him- or herself and/or by the help of an assisting person.
Mattes would call the self-made stretches “active”, but it is contrary to the research
community terminology. A large number of stretches for muscles and parts of muscles
are needed in rehabilitation. AIS can isolate and focus the stretch more precisely than
static stretches. For example, there are 6 AIS stretches for the hamstring muscles
compared to two stretches in static stretching.
Strength training is also needed in rehabilitation and Mattes published in 2006 a book
on strength training (22). The main idea of the book is similar to AIS in focusing strength
exercises on each muscle if possible. During the 4 decades since the 1970s, several
hundred AIS therapists have applied AIS to sport injuries and musculoskeletal
problems. Rehabilitative AIS exercises are based on physiological principles. AIStherapists
have applied AIS in sports teaching, coaching, and assisted stretching. There
is some indication that in elite level sports, where competition is especially hard and
rewards high, AIS has made a breakthrough and many athletes prefer using it. This may
have increased the use of AIS in sports, although there has been a tendency by
athletes to hide the use of AIS in order to be more competitive. AIS therapists have also
been teaching and training AIS to ordinary people. Mattes would suggest that stretching
is almost a daily requirement, as muscles shorten, stiffen, and/or become tense from
67
work, training, and/or stress. In fact, that is one reason he published a book with the title
“Specific Stretching for Everyone” with instructions for 99 AIS stretches (23).
It is interesting and questionable as well that sports medicine research has shown only
so little interest in AIS. At least on the internet pages of the National Academy of Sports
Medicine (NASM) is a text on “Current Concepts in Flexibility Training”, and AIS is one
alternative stretching method (27). Active Isolated Stretches are suggested for warm-up
before sports competitions or high-intensity exercises. But, NASM defines AIS in a
markedly different way than Mattes. Relaxation of the muscle takes place in NASM`s
definition of AIS, but stretching along the stress line of muscle is missing. When
checking videos of the four example stretches of NASM for biceps femoris, quadriceps,
adductors, and pectoral muscles, it appears that they are not consistent with the AIS –
stretches in Mattes books of 2000 and 2012. Both relaxation of muscle and stretching
along the stress line of muscle are required in AIS, and the definition that NASM is
using for AIS is deficient. Without stretching along the stress line of muscle, stretches
are producing less ROM, and also other positive effects of AIS are missing. This may be
one reason, why the medical research community has lost the willingness to study AIS.
CONCLUSIONS
Aaron L Mattes in his many experiments succeeded in finding that stretching along the
line of stress of the muscle and relaxation of the muscle to be stretched in each
repetition of the stretch would diminish the resistance to stretching. Based on this
finding, it has been demonstrated that AIS stretches are performed with less torque than
static stretches of hamstring and calf muscles. Four studies show that AIS would attain
greater or at least the same increases in joint ranges of hamstring muscles as the static
stretches. The findings should be confirmed by additional scientific research.
ACKNOWLEDGMENTS: Special thanks for valuable suggestions concerning the text
to MD, PhD, Associate Professor Katriina Kukkonen-Harjula, Rehabilitation, South
Karelia Social & Health District, Lappeenranta, Finland
Address for correspondence: Dr. Pertti Kukkonen, Espoo, Finland, Email: kohdeven
@gmail.com
Journal of Exercise Physiologyonline
April 2019
Volume 22 Number 2
Editor-in-Chief
Tommy Boone, PhD, MBA
Review Board
Todd Astorino, PhD
Julien Baker, PhD
Steve Brock, PhD
Lance Dalleck, PhD
Eric Goulet, PhD
Robert Gotshall, PhD
Alexander Hutchison, PhD
M. Knight-Maloney, PhD
Len Kravitz, PhD
James Laskin, PhD
Yit Aun Lim, PhD
Lonnie Lowery, PhD
Derek Marks, PhD
Cristine Mermier, PhD
Robert Robergs, PhD
Chantal Vella, PhD
Dale Wagner, PhD
Frank Wyatt, PhD
Ben Zhou, PhD
Official Research Journal
of the American Society of
Exercise Physiologists
ISSN 1097-9751
Official Research Journal
of the American Society
of Exercise Physiologists
ISSN 1097-9751
JEPonline
REFERENCES
1. Alter MJ. Science of Flexibility. (3rd Edition). Human Kinetics, 2004.
2. Ben M, Harvey LA. Regular stretch does not increase muscle extensibility: A
randomized controlled trial, Scand J Med Sci Sports. 2010;20:136-144.
68
3. Blazevich AJ, Cannavan D, Waugh CM, Miller SC, Thorlund JB, Aagaard P, Kay
AD. Range of motion, neuromechanical, and architectural adaptations to plantar
flexor stretch training in humans. J Appl Physiol. 2014;117:452-462.
4. Chan SP, Hong Y, Robinson PD. Flexibility and passive resistance of the
hamstrings of young adults using two different static stretching protocols. Scand
J Med Sci Sports. 2001;11:81-86.
5. Folpp H, Deall S, Harvey LA, Gwinn T. Can apparent increases in muscle
extensibility with regular stretch be explained by changes in tolerance to stretch?
Aust J Physiother. 2006;52:45-50.
6. Freitas SR, Mendes B, LeSant G, Andrade RJ, Nordez A, Milanovic Z. Can
cronic stretching change the muscle-tendon mechanical properties? A review:
Scand J Med Sci Sports. 2018;28:794-806.
7. Gajdosik RL. Effects of static stretching on the maximal length and resistance to
passive stretch of short hamstring muscles, JOSPT. 1991;14:250-255.
8. Gajdosik RL, Linden DWV, McNair PJ, Williams AK, Riggin TJ. Effects of an
eight-week stretching program on the passive-elastic properties and function of
the calf muscles of older women. Clinical Biomechanics. 2005;20:973-983.
9. Gajdosik RL, Allred JD, Gabbert HL, Sonsteng BA. A stretching program
increases the dynamic passive length and passive resistive properties of the calf
muscle-tendon unit of unconditioned younger women. Eur J Appl Physiol. 2007;
99:449-454.
10. Guissard N, Duchateau JA. Effect of static stretch training on neural and
mechanical properties of the human plantar-flexor muscles. Muscle & Nerve.
2004:248-255.
11. LaRoche DP, Connolly DAJ. Effect of stretching on passive muscle tension and
response to eccentric exercise. Amer J Sports Med. 2006;34:1000-1007.
12. Law RYW, Harvey LA, Nicholas MK, Tonkin L, De Sousa M, Finniss DG. Stretch
exercises increase tolerance to stretch in patients with chronic musculoskeletal
pain: A randomized trial. Physical Therapy. 2009;89:1016-1026.
13. Liemohn W, Mazis N, Zhang S. Effect of active isolated and static stretch training
on active straight leg raise performance. Med Sci Sports Exerc. 1999;31(5):
S116.
14. Longo A. Active isolated stretching: An investigation of the mechanical
mechanisms. Brock University: Ontario, Canada, 2009. Internet, June 2018.
69
15. Lopez-Bedoya J, Vernetta-Santana M, Robles-Fuentes A, Ariza-Vargas L. Effect
of three types of flexibility training on active and passive hip range of motion. J
Sports Med Phys Fitness. 2013;53:304-311.
16. Magnusson SP, Simonsen EB, Aagaad P, Dyhre-Poulsen P, McHugh MP, Kjaer
M. Mechanical and physiological responses to stretching with and without
preisometric contraction in human skeletal muscle. Arch Phys Med Rehabil.
1996;77:373-378.
17. Magnusson SP, Simonsen EB, Aagaard P, Sorensen H, Kjaer M. A mechanism
for altered flexibility in human skeletal muscle. J Physiol. 1996;497(1):291-298.
18. Magnusson SP. Passive properties of human skeletal muscle during stretch
maneuvers: A review. Scand J Med Sci Sports. 1998;8:65-77.
19. Marino J, Ramsey JM, Otto RM, Wygand JW. The effects of active isolated vs.
static stretching on flexibility. Med Sci Sports Exerc. 2001;33(5):supplement 1:
S10.
20. Mattes AL. Aaron Mattes` Active Isolated Stretching. Sarasota, Florida, 2000.
21. Mattes AL. Aaron Mattes` Active Isolated Stretching. Sarasota, Florida, 2012.
22. Mattes AL. Active Isolated Strengthening: The Mattes Method. Sarasota,
Florida, 2006.
23. Mattes AL. Specific Stretching for Everyone. Sarasota, Florida, 2000.
24. McMahon T, Catlin P, Cooper A, Fleckenstein D, Maloney P, Marxe R. Effect of
active isolated versus static stretching in asymptomatic adults. J Orthop Phys
Ther. 1997;25(1):Combined Sections Meeting, PO51.
25. Middag TR, Harmer P. Active-isolated stretching is not more effective than static
stretching for increasing hamstring ROM. Med Sci Sports Exerc. 2002;34(5):
Supplement 1, S151.
26. Myers TW. Anatomy Trains, Myofascial Meridians for Manual and Movement
Therapists. (2nd Edition). Elsevier, 2008.
27. National Academy of Sports Medicine (NASM). Current Concepts in Flexibility
Training. (Internet) February 5, 2019.
28. Reid DA, McNair PJ. Passive force, angle, and stiffness changes after stretching
of hamstring muscles. Med Sci Sports Exerc. 2004;36:1944-1948.
70
29. Vernetta-Santana M, Ariza-Vargas L, Robles-Fuentes A, Lopez-Bedoya J. Acute
effect of active isolated stretching technique on range of motion and peak
isometric force. J Sports Med Phys Fitness. 2015;55:1299-1309.
30. Waye D, Mielke A. Active isolated stretching for runners. Peak Running
Performance. May/June, 2010.
31. Weppler CH, Magnusson SP. Increasing muscle extensibility: A matter of
increasing length or modifying sensation? Physical Therapy. 2010;90(3):438-
449.
32. Ylinen J, Kankainen T, Kautiainen H, Rezasoltani A, Kuukkanen T, Häkkinen A.
Effect of stretching on hamstring muscle compliance, J Rehabil Med. 2009;41:
80-84.
Disclaimer
The opinions expressed in JEPonline are those of the authors and are not attributable
to JEPonline, the editorial staff or the ASEP organization.